Types of Heat Exchangers: Advantages, Applications & Design

Heat exchangers are a great piece of sustainable mechanical engineering: they promote energy efficiency by ensuring an optimal heat transfer mechanism.
The various types of heat exchangers we will review in the article utilize different configurations while adhering to the same fundamental principle: to transfer heat from a hot fluid or solid surface to a cooler surface. Therefore the principle is that of "conjugate heat transfer" occurring between two different continua i.e. solid and one or more fluids (we will not deal with direct contact heat exchangers such as in desalination or dehumidification processes while dealing with indirect heat exchangers and presenting a hybrid case).
These versatile components, which can be used in all industries, can also recover and recycle thermal energy.
We will explore different heat exchanger types, coming in various designs and configurations. Each heat exchanger will be presented with its advantages and limitations. We will conclude our review with hints on heat exchanger design methodologies showing examples of Artificial Intelligence engineering applied to the heat exchanger market.

Types of Heat Exchangers
Understanding the different types of heat exchangers is crucial in selecting the right heat exchanger for specific industrial processes, securing efficient heat transfer, and overall system performance.
Double Pipe Heat Exchangers
A double-pipe heat exchanger, or hairpin heat exchanger, consists of two concentric pipes.
In this configuration, one fluid flows through the inner pipe, while the other flows through the outer pipe in the opposite direction. These heat exchangers are simple, compact, and suitable for low to moderate heat transfer applications. They are used in chemical processing plants, small-scale industrial applications, and laboratory equipment.
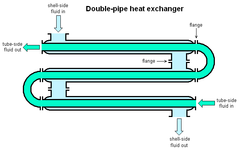
What are the reasons for its popularity in some industries?
Seven Advantages of Double-Pipe Heat Exchangers
- A double-pipe heat exchanger has a compact design, making it suitable for installations where space is limited.
- Double-pipe heat exchangers can handle various fluids, including liquids, gases, and mixtures. This versatility makes them suitable for different applications where various substances must be cooled or heated.
- These heat exchangers offer flexibility in terms of flow configurations. Depending on the process's specific requirements, they can be arranged in parallel or counterflow arrangements. This flexibility allows for optimal heat transfer and efficiency.
- Double-pipe heat exchangers are relatively easy to maintain. The two pipes can be easily accessed for cleaning, inspection, or repairs. This ease of maintenance reduces downtime and ensures the heat exchanger's long-term reliability. Therefore, double-pipe heat exchangers are often cost-effective for small-scale applications since simpler design and construction result in lower manufacturing costs.
- By utilizing a countercurrent flow arrangement, they can achieve a greater temperature differential between the hot and cold fluids, maximizing heat transfer efficiency and providing effective temperature control.
- Double-pipe heat exchangers can be easily scaled up or down to meet the specific requirements of different applications, making them suitable for large chemical processing plants to small laboratory setups.
Shell and Tube Exchangers
Shell and tube exchangers are made of a series of tubes. One set of tubes carries the hot fluid and the other set carries the cold fluid. The tubes are enclosed in a larger cylindrical shell. The shell and tube exchanger configuration is popular in power generation, oil refineries, chemical processing, HVAC systems, food and beverage industry.
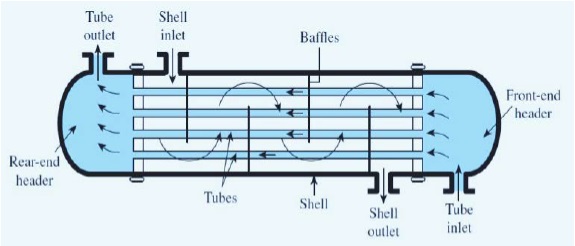
In terms of terminology, the tubes are the smaller diameter pipes within the exchanger where one of the fluids flows. The shell is the outer vessel that holds the tube bundle and through which the second fluid flows.
Other components of the shell and tube setup are the tube sheets, i.e. plates at both ends of the shell where the tubes are fixed, ensuring a tight seal between the shell-side and tube-side fluids; and the baffles, i.e. structures within the shell that direct the flow of the shell-side fluid over the tube bundle to enhance heat transfer and support the tubes.
The shell and tube heat exchanger offers several key advantages that make this heat exchanger type a popular choice in various industrial applications.
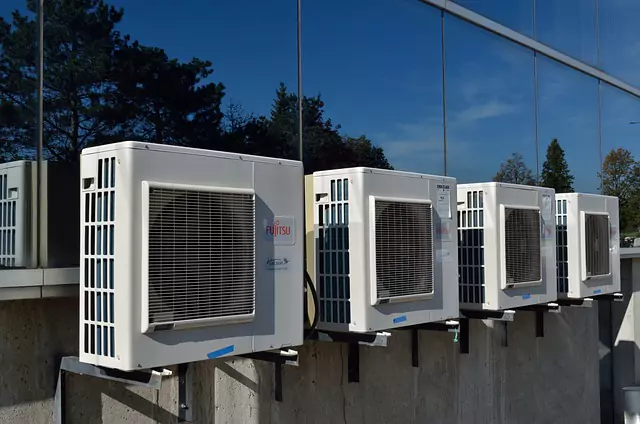
As a first advantage of the shell-and-tube heat exchanger, its high heat transfer efficiency is a result of the design's large surface area provided by multiple smaller diameter tubes within a shell. This design allows for efficient heat exchange between fluids, maximizing the transfer of heat energy and making it particularly effective in managing severe temperature and pressure differentials.
Secondly, the shell-and-tube heat exchanger's versatility and durability are notable. Thanks to its robust construction with stainless steel or other corrosion-resistant alloys, it can handle a wide range of fluids, including corrosive or fouling substances. This construction ensures the exchanger's durability and resistance to harsh operating conditions, making it suitable for demanding industrial environments.
Furthermore, the shell-and-tube heat exchanger offers flexibility in both its design and operation since it can be customized with different tube arrangements, such as straight, U-tube, or multi-pass, and varying shell diameters and lengths to meet specific requirements.
Additionally, the exchanger's modular construction allows for easy maintenance and cleaning, further enhancing its appeal in industrial settings where downtime needs to be minimized.
Finned-Tube Heat Exchangers
A finned-tube heat exchanger is a type of heat exchanger that comprises tubes with external fins attached to them. These fins significantly increase the surface area available for heat transfer, thereby enhancing the heat exchanger's efficiency compared to a version without fins.
This design allows for more effective heat exchange between the fluid inside the tubes and the surrounding air or fluid. Finned-tube heat exchangers' versatility and efficiency make them indispensable in a wide range of industrial and commercial applications, including HVAC systems, radiators, and the aerospace industry. They are commonly employed in HVAC systems to cool or heat air, while in the aerospace industry, they are utilized for thermal management in aircraft and spacecraft.
Additionally, finned-tube heat exchangers are integral components of radiators, where they facilitate the dissipation of heat from the engine coolant to the surrounding air.
Plate Heat Exchangers
Plate heat exchangers are used in HVAC systems, refrigeration, food and beverage industries, chemical processing, and power plants.
A plate heat exchanger employs a series of stacked plates with alternating hot gas and cold fluid passages to facilitate heat exchange. These plates are designed to provide a large surface area for heat transfer, allowing for efficient thermal exchange within a compact structure. This design offers a significant advantage over conventional heat exchangers, as it enables the fluids to contact a much greater surface area due to their distribution across the plates. This configuration promotes enhanced heat transfer and significantly accelerates the rate of temperature change within the system. The figure shows CFD simulations for a herringbone-corrugated plate at different angles of corrugation inclination angle.
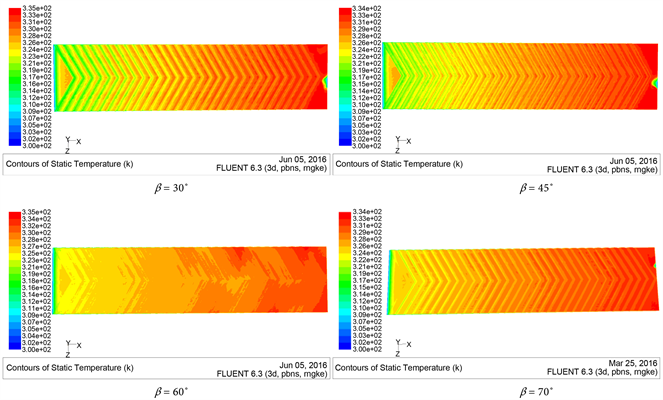
Motivations for Plate Heat Exchangers
First of all, plate heat exchangers are very efficient heat exchangers due to their unique design. They consist of multiple thin plates with corrugated patterns, creating a large surface area for heat exchange. The corrugations enhance turbulence and promote an efficient heat transfer process between the fluids on either side of the plates. This design enables a significant heat transfer coefficient and heat energy in a compact unit, making plate heat exchangers highly efficient for heating or cooling applications.
Plate heat exchangers are known for their compact size and space efficiency. The stacked plate configuration allows for a large HT surface area within a relatively small physical footprint. This compactness makes them ideal for installations where space is limited, such as HVAC systems, refrigeration units, and food processing facilities.
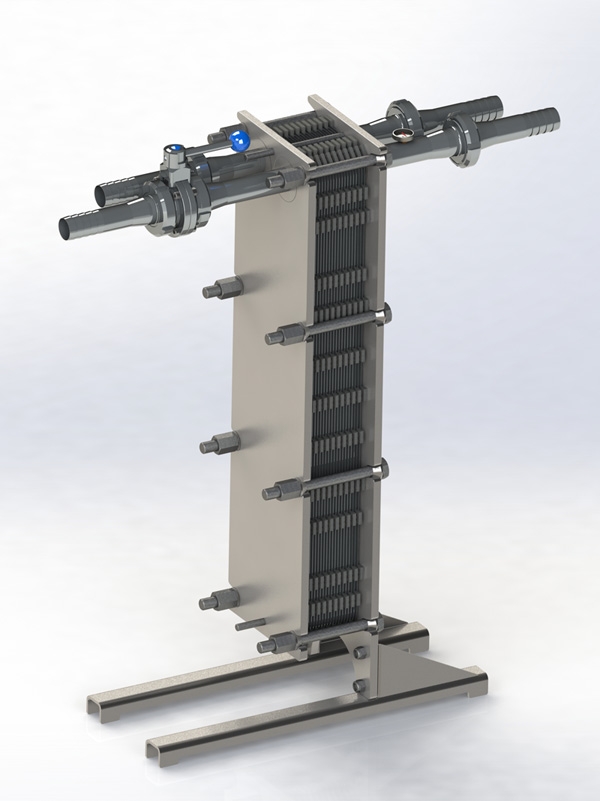
Frame Heat Exchangers
The term "plate heat exchanger" encompasses various designs where heat transfer occurs between thin plates. The frame heat exchanger is one specific configuration within this category.
Plate heat exchangers generally consist of multiple thin plates with alternating channels for the hot and cold fluids to flow through. The fluid channels are separated by the plates, which facilitate efficient heat transfer between the fluids.
The frame heat exchanger is distinguished by the presence of a frame or casing that supports and encloses the plates, providing structural integrity and facilitating fluid connections.
Other Types of Heat Exchangers
We will now briefly review other types of heat exchangers, such as scraper surfaces, the important class of air-cooled heat exchangers and condensers, evaporators, and boilers.
Scraped Surface Heat Exchangers
Scraped surface heat exchangers are designed to handle high-viscosity fluids by continuously removing fouling layers from the heat transfer surface. They offer enhanced heat transfer and prevent product degradation by maintaining consistent temperatures. These exchangers are widely used in the food processing, pharmaceutical, and cosmetics industries.
Air Cooled Heat Exchanger
Air-cooled heat exchangers utilize ambient air to cool fluids, making it energy-efficient and environmentally friendly. Due to its simple design and fewer moving parts, it requires little maintenance. These exchangers are commonly used in the power generation, petrochemical, and refrigeration industries.
Classification of Heat Exchangers by Flow Type
We will review the different types of flow configurations for heat exchangers.
Parallel Flow Heat Exchangers
In this type of heat exchanger, fluids flow in the same direction within the tubes, which can result in less efficient heat transfer compared to counterflow configurations. However, "parallel" heat exchangers are often simpler in design and can be more compact. They are commonly used in applications where space is limited.

Cross Flow Heat Exchangers
Here, each fluid flows perpendicular to the other, with one fluid passing through the tubes and the other passing over the external surface of the tubes. This configuration allows for efficient heat transfer across the exchanger's surface. It is commonly used in applications such as air conditioning systems, where one fluid is a gas and the other is a liquid. The crossflow heat exchanger offers a balance between thermal efficiency and compact design.
Counter Flow Heat Exchangers
In these types of heat exchangers, the two fluids flow in opposite directions. This design maximizes the temperature difference between the fluids along their entire flow paths, allowing to transfer heat even more efficiently than in the previous configurations.
Heat Recovery and Heat Exchangers
Various industrial processes and energy systems often generate significant waste heat. Regenerative heat exchangers are designed to recover and transfer this waste heat to another medium, such as water or air, for reuse in other applications.
Regenerative exchangers utilize both indirect and direct heat transfer principles to achieve high thermal efficiency.
Heat Recovery Use Cases
We talked about sustainable engineering and here we have three big examples. For instance, waste energy recovery from data centers exemplifies a practical application of energy efficiency and sustainability principles, demonstrating how waste heat can benefit local communities and contribute to a more resilient and environmentally conscious energy infrastructure.
(1) In an industrial facility where machinery generates substantial heat, instead of letting thermal energy dissipate into the air, a recovery system can capture it. Regenerative heat exchangers can transfer the excess heat from the machinery to water. This heated water can then be used for processes like pre-heating feedwater for boilers, space heating, or hot water supply systems.
(2) Another practical example is data centers. These facilities run servers that produce a lot of heat. By using thermal energy recovery systems, waste heat can be captured and redirected to heat nearby buildings or provide hot water for nearby communities.
(3) In residential applications, energy recovery systems can take the heat from exhaust air and transfer it to the incoming fresh air, reducing energy consumption but also maintaining a comfortable indoor temperature.
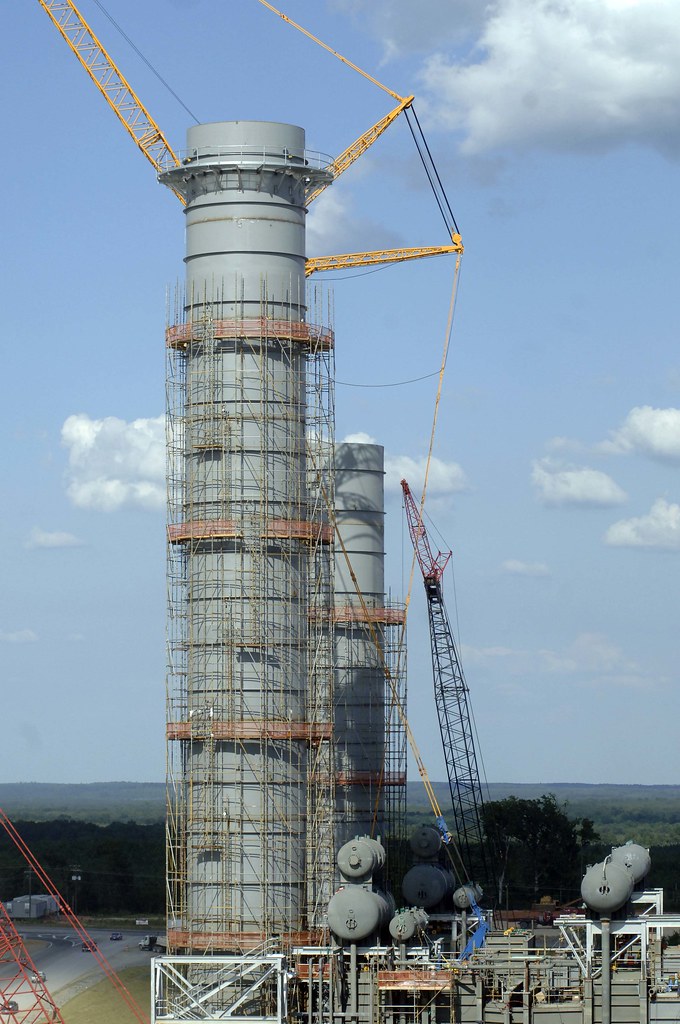
Hybrid Characteristics
The hybrid characteristics of this type of heat exchanger is due to direct and indirect trasnfer. Heat trasnfer between the fluid and the solid matrix occurs indirectly through conduction, making it an indirect heat exchange process. In addition to indirect heat transfer, regenerative heat exchangers also utilize direct heat transfer principles during the transient periods when hot and cold fluids are in contact with the solid matrix simultaneously. This allows for more efficient heat exchange between the fluids and the solid material.
How Do Heat Exchangers Operate?
Heat exchangers are thermodynamic devices that enable efficient heat transfer between fluids or between a fluid and a solid surface: they operate based on several thermodynamic principles and equations we will review below.
Thermodynamics Principles of Heat Exchangers
Let us start with key concepts using simple maths. A fundamental equation governing heat transfer (HT) Q is Newton's Law of Cooling, given by Q = h × S × ΔT where h is the overall heat transfer coefficient, and S is the surface area available.
The overall heat transfer coefficient h considers various resistances to heat transfer, such as conduction, convection, and radiation. It depends on the materials' thermal conductivities, the walls' thickness, and the fluids' flow rates.
Heat exchangers employ the principle of energy conservation, represented by the first law of thermodynamics. This law states that the energy entering a system must equal the energy leaving it. In the case of a heat exchanger, the energy transferred as heat from the hot fluid equals the energy gained by the cold fluid.
Different types of heat exchangers utilize specific flow patterns to optimize heat transfer. For example, in a parallel flow heat exchanger, both fluids enter at one end, and hot and cold liquids travel parallel to each other, resulting in a gradual ΔT reduction along the length of the exchanger. In a counterflow heat exchanger, the hot and cold fluids enter opposite ends, maximizing the ΔT and enhancing transfer.
Effectiveness
Efficiency is a crucial consideration in heat exchanger operations. The effectiveness ε of a heat exchanger is defined as the ratio of actual HT to the maximum possible HT. It can be calculated using the equation ε = Qₐ / Qₘₐₓ where Qₐ is the actual HT observed, and Qₘₐₓ is the maximum transfer of heat possible based on the ΔT between the fluids.
Engineers consider parameters such as fluid flow rates, properties, and design features to optimize heat exchanger performance. Increasing surface area, using fins or turbulators, and employing materials with high thermal conductivity can enhance heat transfer.
Alternative Heat Exchanger Materials
- Ceramics are highly effective for applications involving high temperatures that melt metals like copper. They are also popular for their ability to handle corrosive and abrasive fluids.
- Plastics are known for their lighter weight and lower cost compared to metals. They are resistant to corrosion and fouling and can be engineered to possess good thermal conductivity. However, they are often susceptible to mechanical weakness and degradation over time. While generally not suitable for high-temperature applications, plastic heat exchangers can be a suitable choice for scenarios operating at everyday temperatures.
- Composite heat exchangers leverage the best properties of their parent materials, combining metals' high thermal conductivity with plastics' reduced weight and superior corrosion resistance.
- Carbon Nanotubes: Looking ahead, there is potential for the use of even more innovative materials. For instance, carbon nanotubes, which consist of thin hexagonal carbon sheets wrapped to form pipes, exhibit remarkable heat-conducting properties. Nanotubes exhibit extraordinary mechanical strength, stiffness, and conductivity due to their atomic structure. One of the most remarkable properties of carbon nanotubes is their exceptional thermal conductivity. They can efficiently conduct heat along their length, making them ideal candidates for thermal management applications.
Heat Exchanger Simulation
The conduction equation is commonly used in heat exchanger simulations to model heat exchange within a solid medium. It describes how heat is conducted through a material based on its temperature distribution. Solving the conduction equation allows us to determine the temperature profile and the HT rates within a heat exchanger. Designers should be empowered to access simulations without hindrances or requirements for massive cloud computing structures: we will see how AI can provide an answer for product designers.
Analytical Solution for Heat Exchangers
Determining the temperature distribution within a heat exchanger involves searching for an accurate mathematical expression known as the analytical solution of the conduction equation. These solutions, however, are only viable for simple geometries and boundary conditions. The complexity of the heat exchanger geometry or boundary conditions in practical cases often renders analytical solutions unattainable. Analytical solutions can be derived for simple geometries, such as a 1D heat conduction problem in a rod.
In scenarios where the heat exchanger exhibits more intricate geometries and boundary conditions, numerical methods are employed to solve the conduction equation. These numerical methods provide comprehensive solutions for 1D, 2D, or 3D heat exchange processes, taking into account time dependence. By utilizing numerical methods, engineers can gain a deeper understanding of the heat transfer process within the system, allowing for more complex geometries and boundary conditions to be accurately addressed.
These methods involve discretizing objects by meshing them (see figure) and approximating the temperature at each point within the computational mesh.
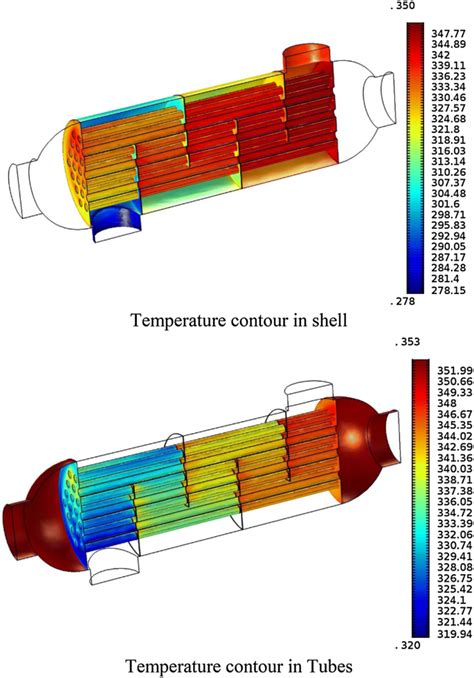
The most widely used numerical methods for solving the conduction equation include the Finite Difference Method (FDM), Finite Element Method (FEM), and Finite Volume Method (FVM).
AI for Heat Exchangers
Various industries have stored their simulation histories in the company's PLM (Product lifecycle management). However, can these data be utilized more productively besides storing and retrieving them?
Shape Optimization for Heat Exchangers
AI helps with a data-driven approach that can simulate various heat exchanger with machine learning optimization. It uses deep learning to understand the connection between shape (CAD) and functionality (CFD, FEA), making custom surrogates for the CFD or FEA solvers. Comparing AI, CFD, or FEA results shows promising outcomes for the tested sample.

The solution is particularly suitable for design engineers and anyone wishing to operate on heat exchanger geometries to optimize their performance. The outcome can be a generatively designed heat exchanger, as in the figure.

Conclusion
In our thorough investigation, we analyzed different types of heat exchangers and their wide-ranging applications. Furthermore, we explored various techniques to extend the durability of these devices. At the heart of our article lies a detailed classification of heat exchanger types based on their geometric and flow configurations.
We also investigated design resources for these devices and briefly discussed Deep Learning applications to mechanical engineering. This AI technology has shown promising results in analyzing and enhancing heat exchangers.
Stay tuned for more articles on this subject in the future... it's still hot!