What Is Thermal Management? Challenges and Solutions
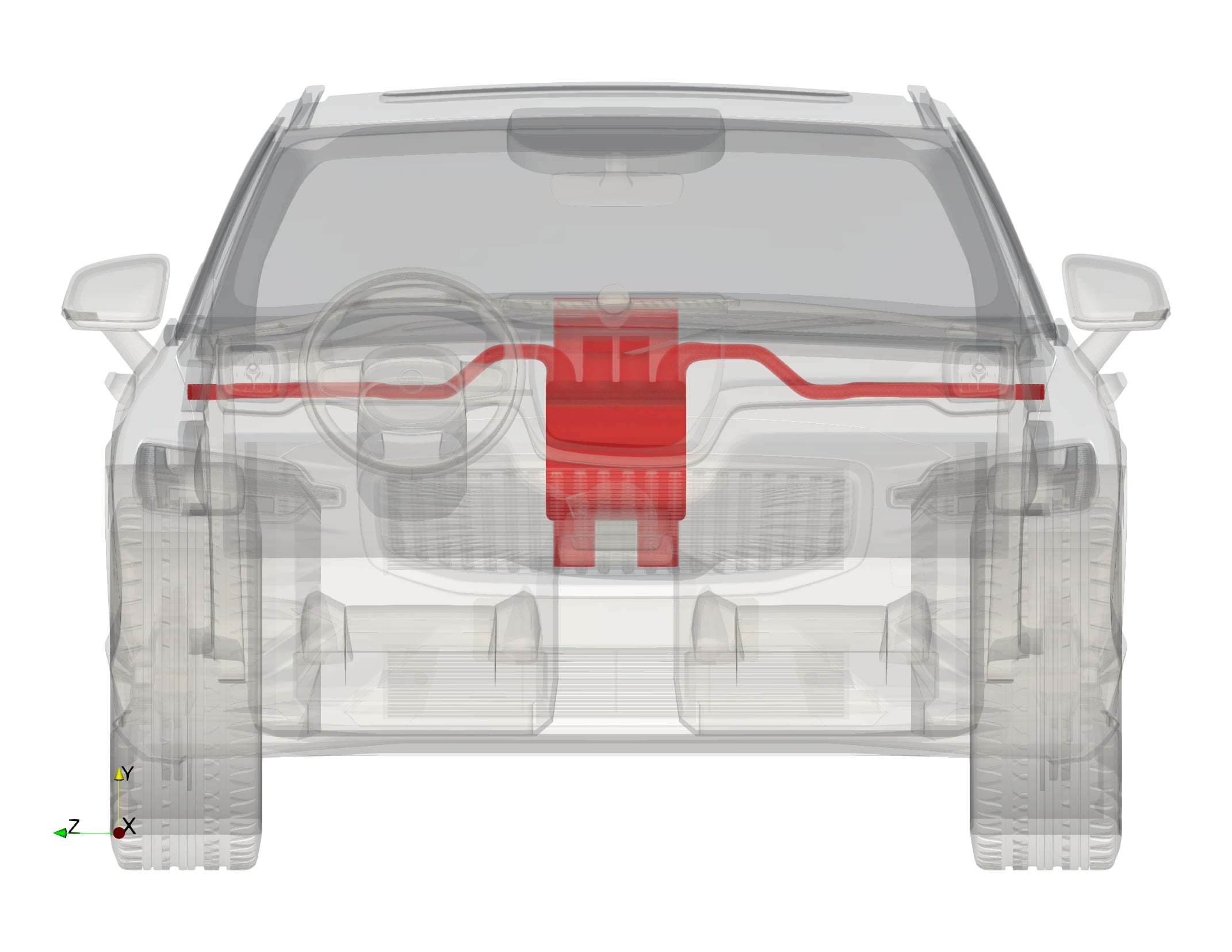
The demand for robust and efficient devices is constantly increasing. For example, our cars are assemblies of components that are becoming smaller and smaller, while we expect them to perform even better. How can a heat source be managed in an ever-smaller environment? This is the main challenge for thermal management solutions: the solution to prevent a heat source from damaging a device is to remove heat via conduction convection and radiation sinks.
The world would not be the same without thermal management solutions—most of your devices would not last more than a few minutes without them. Even when passively waiting for a cryptocurrency to increase in value, you should be aware that cryptocurrency mining is an energy-intensive operation since data centers require a lot of heat dissipation. Therefore, thermal management is critical to ensuring optimal device performance and longevity for visible and invisible (remote) devices. Designing a thermal management solution that optimally cools electronic devices highlights just one industry sector that needs proper thermal management.
With challenges like the above, engineers are asked to design thermal management solutions that can effectively dissipate heat from increasingly compact devices without compromising performance or reliability. This requires innovative approaches such as advanced materials, enhanced heat transfer techniques, and sophisticated cooling systems.
Innovation and research address challenges that require thermal management systems, such as excess heat, varying temperature thresholds, and efficient heat dissipation.
The article will focus on the challenging and pervasive world of Thermal Management and a key component of thermal management solutions- the heat exchanger. Various types of heat exchangers play a role in thermal management.
We will also explore thermal management simulation, focusing on engineers' challenges and examining innovative solutions.
One of the most innovative solutions is to leverage Artificial Intelligence to optimize heat exchangers.
What is a Thermal Management System?
Thermal management involves strategies and techniques for controlling and regulating the temperature of electronic devices, other mechanical processes and systems, and various components. This ensures optimal performance, reliability, and longevity by dissipating excess heat, preventing overheating, and maintaining stable operating conditions through heat sinks, fans, thermal interface materials, and liquid cooling systems.
The Impact of Thermal Management
Thermal management systems are crucial in keeping equipment cool and preventing overheating. These systems are designed to manage the transfer, dissipation, and regulation of heat generated by electronics during operation. Effective cooling of electronic devices ensures optimal performance and reliability. Additionally, air cooling systems in buildings help dissipate heat.
Mechanisms of Heat Transfer Process
We will briefly review the mechanisms of conduction, convection, and radiation.
Thermal Conductivity
Thermal conductivity is a fundamental material property that measures their ability to conduct heat. It represents the rate at which heat is transferred through a material in response to a temperature difference. Materials with high thermal conductivity, such as metals, are exceptionally effective at transferring heat, making them well-suited for applications like heat sinks and thermal interface materials. On the other hand, materials with low thermal conductivity, such as wood or foam, serve as good insulators, impeding heat flow.
The following equation (a 1-Dimensional steady Fourier equation) quantifies how heat "Q" is conducted through a material, with thermal conductivity "𝑘" playing a central role in determining the rate of heat transfer and showing that materials with higher thermal conductivity values allow heat to transfer more quickly through them. Materials with lower values contrast heat flow: Q = - 𝑘 A ΔT / L.
Convection
Heat transfer through convection is accomplished by moving fluids such as liquids or gases. This process occurs when a fluid is heated, causing it to become less dense and rise, while cooler, denser fluid sinks, thus creating a continuous cycle of movement.
Convection is an efficient method of transferring heat in fluids because it combines the fluid's thermal conductivity with its movement, resulting in an enhanced overall heat transfer rate.
Natural Convection
Natural convection occurs when a fluid, such as air or water, is heated by a nearby heat source, causing it to expand and become less dense. The less dense fluid rises while cooler, denser fluid sinks, creating a natural circulation pattern. An example of natural convection is the rising of warm air near a heater or radiator, which creates air currents in a room.

Forced Convection
In forced convection, a fluid is circulated by an external force such as a fan or a pump. This enhances heat transfer by increasing the fluid velocity and promoting mixing.
Examples include fans in air conditioning systems to distribute cooled air and coolant circulation in automotive engines to remove heat from the engine block.
Radiation
Radiation is the process by which heat is transferred through the emission of electromagnetic waves, particularly in the infrared spectrum. Unlike conduction and convection, radiation does not rely on a medium to propagate and can occur even in a vacuum. This heat transfer method is particularly effective over vast distances and in environments where conduction and convection are limited, such as in the vacuum of space.
Examples are:
- Solar radiation: Heat from the Sun warming the Earth
- Infrared heaters: Devices that emit infrared radiation to heat objects directly in their path
After reviewing the basic physics of conduction, convection, and radiation, we will move on to the heat sink as a core concept in thermal management solutions.
Thermal Management Techniques: Heat Sink
Heat sinks are among the critical components in thermal management.
The heat sink is a passive cooling device that absorbs and dissipates excess heat.
In electronic components and other systems, the heat sink acts as a thermal bridge, transferring thermal energy from the heat source to a medium, often the surrounding air or a cooling fluid.
Heat sinks are usually made of materials with high thermal conductivity, and their design includes fins or other structures to maximize their surface area for heat transfer and, hence, dissipation.
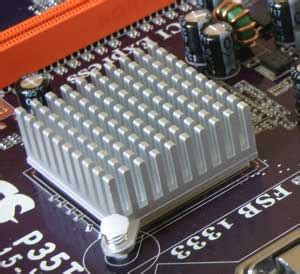
A heat sink is a thermal management system that enhances heat transfer by increasing heat density over its surface area. It dissipates heat from vital electronic components and other mission-critical components to ambient temperature.
Challenges of Thermal Management
Thermal management faces several challenges in maintaining optimal operating temperatures.
One of the primary challenges in aerospace or automotive electronics is dissipating heat when dealing with the ever-increasing heat densities they generate. As devices become more powerful and compact, they generate more heat within a limited space.
Without effective thermal management, this excess heat can accumulate and cause temperature spikes, leading to performance degradation, reduced lifespan, and even device failures. How to enhance thermal transfer?
Thermal management techniques face a challenge due to the different temperature thresholds of various configurations within a system. Each component may have distinct temperature limits for optimal performance and longevity. Therefore, thermal management must be carefully designed to meet the specific thermal requirements of each element, ensuring they operate within their designated temperature ranges and avoiding overheating sensitive components.
Thermal Management Technologies
Engineers have developed various thermal management techniques to overcome the above challenges.
Thermal Management Solutions: Heat Pipes
Among these solutions, heat pipes have proven highly effective as a thermal management and cooling system part. Heat pipes efficiently transfer heat using the principles of phase change and capillary action.
These sealed, hollow pipes contain a working fluid that evaporates at the heat source, travels along the heat pipe, and condenses at the cooler end. This process enables rapid thermal energy transfer, making heat pipes ideal for high-dissipation applications.
Thermal Management Solutions: Thermal Interface Material
Another vital component in thermal management is the thermal interface material (TIM). TIMs are substances between the heat source, such as a microchip and the heat sink, that improve thermal conductivity and fill microscopic air gaps, transferring heat.
These materials enhance the contact between the two surfaces, reducing thermal resistance and facilitating efficient heat transfer.

Liquid Cooling for Heat Dissipation
Liquid cooling systems have also emerged as a popular solution for thermal management, particularly in high-power applications. These systems utilize liquid coolants, such as water or specialized fluids, to absorb and carry away heat from electronics as heat sinks.
Liquid cooling systems offer superior heat removal and dissipation capabilities compared to air cooling, making them ideal for applications that demand efficient cooling.
Air Cooling System for Heat Dissipation
Air cooling remains the basis of widely used and cost-effective thermal management solutions. Air cooling systems employ fans or blowers to create airflow, facilitating convective heat transfer.
As cooler air passes over the heat sink and warmer device, it absorbs the excess heat, reducing the device's temperature. While cooling by air may not match the cooling efficiency of liquid cooling, this thermal management system remains a viable option for many electronic parts, especially those with lower power densities.

Thermal Management in Motor Drives
A motor drive, also known as a motor controller or inverter, is an electronic device that controls an electric motor's speed, torque, and direction. It converts electrical power from a DC or AC power source into the specific voltage and frequency required to drive the motor.
Thermal management is crucial in motor drives due to the significant heat generated during operation. This heat primarily originates from power losses in semiconductor devices (such as MOSFETs or IGBTs) and other components within the motor drive. Excessive heat can degrade the performance and reliability of the motor drive, leading to reduced efficiency, shortened lifespan, and potential failure of critical components. Therefore, effective thermal management is essential to dissipate heat efficiently and maintain optimal operating conditions for the motor drive.
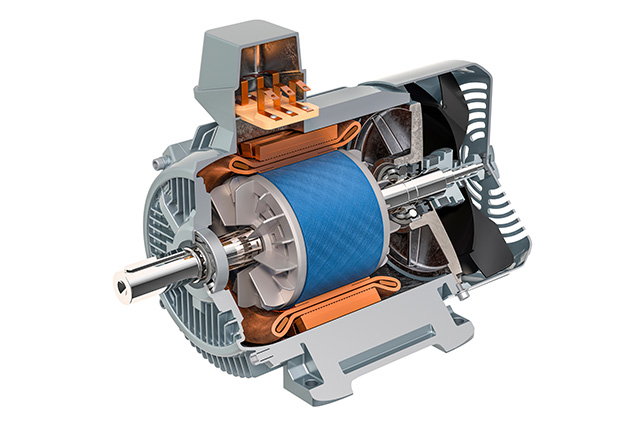
How Can We Manage Heat in Motor Drives?
Heat sink design is essential for heat dissipation from the power electronics components. Heat sinks with suitable size, shape, and material properties increase the surface area for heat transfer and provide pathways for convective cooling. Finned heat sinks are commonly used to enhance heat dissipation in motor drives.
Thermal interface materials, such as grease, pads, or phase-change materials, improve the thermal contact between the semiconductor devices and the heat sink. These materials fill the microscopic gaps between mating surfaces, reducing thermal resistance and enhancing heat transfer efficiency.
Fans or blowers are active cooling systems that augment heat dissipation in motor drives. Forced-air cooling helps remove heat from heat sinks and other components, especially in enclosed or high-temperature environments. Alternatively, liquid cooling systems circulate coolant through channels or heat exchangers, providing efficient cooling for high-power motor drives.
Proper design of the motor drive enclosure is essential for effective thermal management. Enclosures should incorporate adequate ventilation or cooling provisions to prevent heat buildup. Optimizing airflow and component placement within the enclosure helps maintain optimal operating temperatures and prevent overheating.
GPU and Thermal Management Solutions
Because of their impact, GPUs' importance cannot be overestimated. We will first investigate a few GPU applications before tackling how to cool them.
Graphics Rendering
GPUs are primarily known for rendering high-quality graphics for video games, movies, design software, and virtual reality applications. They process complex graphical data quickly and efficiently, delivering smooth, immersive visual experiences.

Parallel Processing Power
CPUs excel at parallel processing, allowing them to perform multiple computations simultaneously. This capability is leveraged in scientific computing, artificial intelligence, deep learning, and data analytics, where massive parallelism significantly accelerates computation-intensive tasks. For example, CFD (Computational Fluid Dynamics) has benefited immensely from GPU acceleration in terms of scientific and engineering computing. CFD simulations are crucial for understanding fluid flow and thermal management. CFD involves solving complex mathematical equations that describe the behavior of fluids. These simulations are computationally intensive and require significant time when performed on CPU-based systems. The parallel processing power of GPUs has revolutionized this field by enabling much faster computations. A significant (at least 1 order of magnitude) reduction in computation time allows engineers to run more detailed and accurate simulations, leading to better design and optimization of cooling systems.
Acceleration of AI and Machine Learning
GPUs have become indispensable for accelerating AI and machine learning algorithms, including neural networks and deep learning models. Their highly parallel architecture enables rapid training and inference, leading to breakthroughs in image recognition, natural language processing, and autonomous vehicles. GPUs allow for the efficient processing of vast amounts of data by performing multiple operations simultaneously. This speeds up the most essential computational tasks for AI development. This acceleration has enabled researchers and engineers to iterate faster, experiment with more complex models, and achieve higher accuracy rates in their AI applications. Furthermore, the scalability of GPUs supports the deployment of AI models in real-time systems, enhancing their applicability in fields such as healthcare diagnostics, financial forecasting, and personalized recommendations. The role of GPUs in pushing the boundaries of what is possible in machine learning and artificial intelligence.
Cryptocurrency Mining
GPUs are commonly used in cryptocurrency mining rigs to perform the complex mathematical calculations required to validate and secure transactions on blockchain networks. Their computational power and efficiency make them well-suited for this task, although their use in mining has led to increased demand and scarcity in the consumer market.
Why Cool GPUs
Due to their high power consumption and intensive computational tasks, GPUs generate heat during operation. This heat can accumulate without effective cooling, leading to thermal throttling, reduced performance, and potential damage to the GPU components.
Maintaining optimal operating temperatures is essential for maximizing GPU performance. Cooling solutions help dissipate heat efficiently, allowing the GPU to operate at its full potential without throttling. This is particularly crucial for demanding tasks such as gaming, 3D rendering, and AI training, where consistent performance is critical.
Excessive heat can degrade the lifespan and reliability of GPU components, including the graphics processor, memory chips, and voltage regulators. Proper cooling helps mitigate thermal and mechanical stress together, prolonging the lifespan of the GPU and reducing the risk of premature failure.
How to Cool GPUs
Cooling a GPU effectively involves a combination of built-in solutions, aftermarket upgrades, and optimizing case airflow. Advanced cooling techniques, such as liquid and hybrid solutions, provide superior thermal management for high-performance GPUs, ensuring they operate efficiently and reliably under heavy loads.
Built-In Cooling Solutions - Standard Air Cooling System
Most consumer GPUs come equipped with built-in fans and heatsinks. The heatsink absorbs heat from the GPU die, while the fans actively dissipate this heat by moving air across the heatsink’s surface. This is usually sufficient for mainstream GPUs under typical operating conditions. Some GPUs feature direct contact heat pipes that enhance heat transfer efficiency from the GPU die to the heatsink, improving cooling performance.
Aftermarket Air Cooling Solutions
Aftermarket GPU coolers often incorporate larger heatsinks and multiple fans to improve heat dissipation. These coolers are designed to replace the stock cooling solution and enhance thermal management.
High-quality Thermal Interface Materials, such as thermal paste or pads, are applied between the GPU die and the heatsink. Upgrading to a premium Thermal Interface Material can reduce thermal resistance and improve heat transfer efficiency.
Case Airflow Optimization
Effective GPU cooling is also dependent on overall case airflow. Ensuring adequate airflow within the PC case involves strategically placing case fans to promote efficient air circulation.
Installing additional intake and exhaust fans in a balanced manner can create positive air pressure, which helps expel hot air from the case more effectively and reduces dust accumulation inside the case.
Keeping the case's interior tidy by managing cables and removing unnecessary components can enhance airflow. Proper cable management minimizes obstructions that can impede air movement.
Advanced Cooling Solutions for GPUs
High-end GPUs may come with integrated liquid cooling solutions like pre-filled closed-loop liquid coolers. These systems include a pump, a radiator, and one or more fans. Liquid cooling provides superior thermal performance by transferring heat away from the GPU die to the radiator, where it is dissipated by the fans.
Enthusiasts and professionals often opt for custom liquid cooling loops, allowing more extensive cooling configurations. These setups can include multiple radiators, water blocks for the GPU, CPU, and other components, and high-flow pumps to ensure efficient heat dissipation.
The choice of coolant in liquid cooling systems can also impact performance. Coolants with higher thermal conductivity can enhance heat transfer efficiency.
Hybrid GPU Cooling Solutions
Some GPU solutions combine air and liquid cooling technologies. For example, a GPU might use a liquid cooling block for the GPU die while retaining air cooling for other components, such as VRMs (Voltage Regulator Modules) and memory chips.
Advanced GPU coolers may also include active backplate cooling, where additional heat sinks and fans are mounted on the backplate to dissipate heat from the GPU's rear side.
Thermal Management Solutions via Simulation
In engineering, where innovation and precision go hand in hand, the design and optimization of thermal management systems are critical for ensuring efficient and reliable operations. Engineers employ tools and techniques to achieve optimal thermal performance, such as Computer-Aided Design (CAD) and advanced 3D simulations for heat transfer.
This section will explore the role of CAD and simulation techniques in thermal management, their benefits, and how they contribute to designing and analyzing effective thermal management solutions.
CAD and Simulation
In thermal management, CAD and simulation techniques provide engineers with powerful tools for designing, optimizing, and analyzing thermal management. CAD enables the precise creation and visualization of thermal components, while simulations allow for detailed thermal energy transfer behavior and temperature distribution analysis.
Integrating CAD and simulations facilitates iterative design cycles, enhancing thermal performance and efficient heat dissipation.
With these tools, engineers can meet the ever-increasing demands of thermal management in electronics and various other industries.
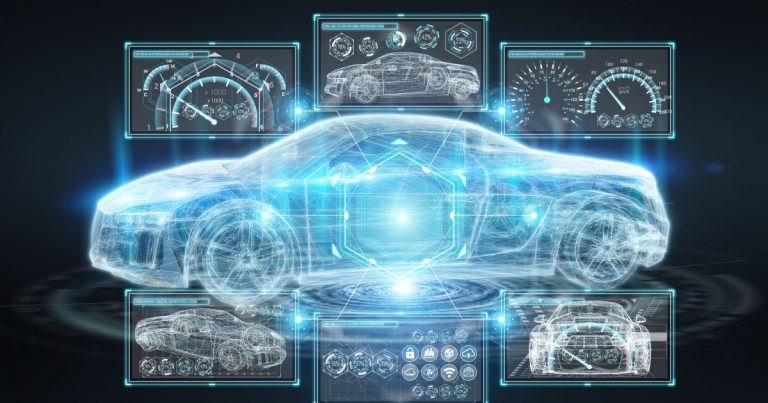
Understanding CAD for Thermal Management Systems
Computer-aided design (CAD) is a fundamental tool in modern engineering. It enables the creation, modification, and optimization of complex designs with utmost accuracy. In thermal management, CAD software allows engineers to design and visualize the components and systems involved in heat dissipation.
CAD provides a digital environment where engineers can create and manipulate 3D models of heat sinks, enclosures, and other thermal management elements. CAD software streamlines the design process, facilitating precise geometry definition, material selection, and overall system integration.
Benefits of CAD in Thermal Management Systems
CAD brings numerous benefits to the field of thermal management. Engineers can use CAD software to explore design iterations and evaluate their impact on thermal performance. They can analyze heat sink geometries, fin arrangements, and fluid flow patterns to optimize heat dissipation.
CAD tools enable the evaluation of thermal resistance, fluid dynamics, and other critical parameters, allowing engineers to fine-tune their designs for enhanced heat transfer efficiency. Furthermore, CAD models serve as a basis for prototyping and manufacturing, ensuring seamless integration of thermal management components into the overall system.
Simulation Techniques
In addition to CAD, advanced simulation techniques are pivotal in designing and analyzing thermal management systems. Thermal simulations enable engineers to predict and evaluate electronics' heat transfer behavior, temperature distributions, and thermal stresses.
Finite Element Analysis (FEA) and Computational Fluid Dynamics (CFD) simulations are widely utilized in engineering to model and analyze systems' thermal behavior.
FEA is particularly effective in studying structural and thermal responses, while CFD is used to analyze fluid flow and heat transfer. These simulations allow engineers to understand how a system responds to thermal loads and operating conditions, enabling them to optimize designs and make informed decisions about thermal management strategies.
Engineers can assess a system's thermal performance and identify potential areas for improvement by simulating scenarios ranging from steady-state to transient conditions. This detailed analysis ensures systems operate within safe temperature limits and perform optimally in real-world applications.
Benefits of Simulation in Thermal Management
Simulation offers several advantages to thermal management.
Engineering simulation in 3D (CAE) allows engineers to understand heat dissipation and temperature control for electronics comprehensively.
Through simulations, engineers can identify potential hotspots, optimize airflow, and assess the effectiveness of heat sink designs. Simulations also provide a cost-effective alternative to physical prototyping and testing, saving time and resources in the design process.
As we said before, engineers can explore different operating conditions, evaluate thermal performance, and identify potential issues before manufacturing the system via simulation. This simulation of different scenarios is known as "design space exploration." If objective targets and constraints can be set, it is, in principle, possible to exploit optimization algorithms to go beyond simple verification and proactively elaborate new shapes of heat exchangers.
Integration of CAD and Simulation
The true power of a thermal management solution lies in the integration of CAD and simulation techniques. CAD models are the foundation for accurate simulations, providing geometry, material and thermal properties, and boundary conditions necessary for accurate analysis.
The seamless integration of CAD and simulation tools enables engineers to validate their designs, optimize thermal performance, and ensure the thermal management system meets the desired specifications. This integration allows for iterative design cycles, where changes in the CAD model can be quickly evaluated through simulations, resulting in refined and efficient thermal management products and solutions.
Real-World Applications of CAD and Simulation Techniques
CAD and simulation techniques are widespread in various industries and applications. In electronics, thermal management is crucial for optimizing the performance and reliability of devices such as smartphones, laptops, and server racks. Engineers can design effective cooling solutions using CAD and simulations, select appropriate heat sink configurations, and optimize airflow to prevent overheating.
Other areas that benefit from CAD and simulation techniques for thermal management include automotive engineering, where maximum efficiency and heat dissipation is critical for electric vehicles, HVAC system design and internal combustion engines, and aerospace engineering, where proper thermal management ensures the safe operation of electronic systems in extreme temperature environments.
Power generation, consumer electronics, and industrial machinery also use CAD and simulations to design efficient cooling solutions and regulate temperature control.

The Emergence of AI: New Frontier in Thermal Management
Artificial intelligence (AI) has emerged as a powerful tool in various industries.
In thermal management, engineers face complex challenges that require sophisticated solutions, and AI has proven to be one of the most promising.
AI offers great opportunities for optimizing thermal management systems by analyzing large amounts of data, recognizing patterns, and helping engineers make intelligent decisions. This section explores the increasing impact of AI in thermal management, its potential applications, and the benefits it provides to engineers in this field, including the primary benefits of AI-powered analytics and optimization.
The innovation of AI in thermal management lies in its ability to use advanced analytics and optimization algorithms. By employing machine learning techniques, AI algorithms can process large volumes of data related to heat dissipation, temperature profiles, and system performance. This allows engineers to gain deep insights into thermal behavior, identify patterns, and make data-driven decisions to enhance the efficiency of thermal management systems.
The ultimate step in AI implementation is the generative design approach.
Conclusion
We have learned that thermal management is a crucial aspect of modern engineering. By exploring various technologies, such as air or liquid cooling systems and advanced digital threads with Simulation and AI, we better understand how to effectively manage essential components in our everyday lives.
Thermal management systems, backed by technologies like heat sinks, heat pipes, and advanced cooling methods outlined in the article, play a vital role in maintaining optimal operating temperatures. They also ensure the reliability and longevity of electronic components.