The Role of AI in Transforming Structural Engineering
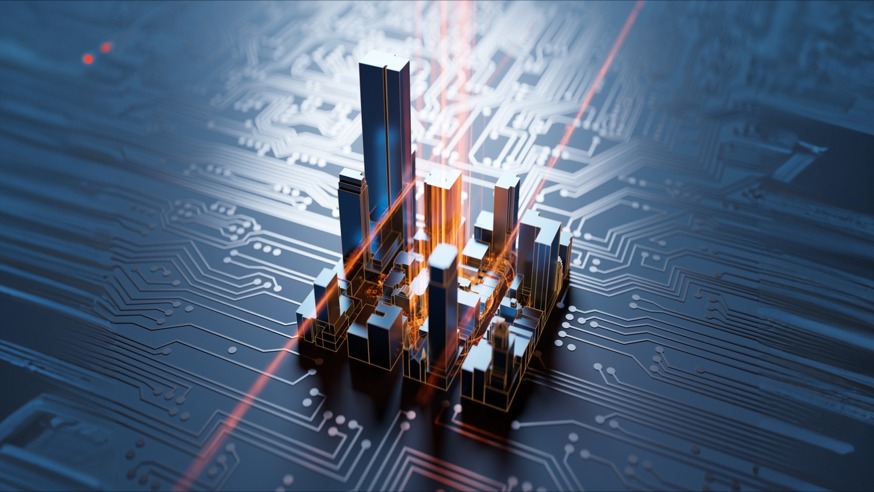
Can AI help structural engineers?
Let us take a look at simulation software for several sectors ranging from automotive to civil and environmental engineering. We will also sum up AI in structural engineering design. Also, we will focus on the potential impact on teams involved in the design process and R&D analysis processes related to structural engineering and structural health monitoring.
Structural engineering encompasses a diverse range of activities and applications to ensure infrastructure safety and functionality. Structural engineers use their expertise and advanced tools from structural analysis (e.g. FEA software) to design optimization. This allows them to tackle complex challenges and deliver innovative solutions that shape the built environment, transportation, and daily objects. Software technology continues to evolve, and the role of structural engineering will remain indispensable in the AI age in creating resilient, sustainable, and aesthetically pleasing structures.
Artificial Intelligence offers the potential to help structural engineering practices, but it is important to approach its integration with caution and critical scrutiny. By taking into account the complementary roles of AI-driven methodologies and human expertise (including in human expertise physics-driven solvers such as FEA software), engineers can leverage the benefits of Artificial Intelligence while mitigating potential risks and ensuring the integrity and reliability of structural designs.
Moreover, the Deep Learning approach to structural engineering AI presented here allows the democratization of simulation software for many more corporate levels than usual, including all engineers or architects who have no specialist skill in FEA but can obtain FEA data from the experts.
The development of validation techniques is essential for evaluating the precision, accuracy, and performance of AI-driven approaches in structural analysis and design.
Structural Engineering and Structural Design
Structural engineering involves the application of scientific and engineering principles to design, analyze, and construct various types of structures, including buildings, bridges, dams and towers. Structural engineers leverage their expertise in mechanics, materials science, and mathematics to address complex challenges and deliver more innovative designs and solutions that meet the needs of society.
Structural engineering is nowadays essential in engineering disciplines such as civil engineering. It encompasses an array of activities ensuring infrastructure stability, safety, and functionality. Structural engineers shape the built environment through all the phases of planning, analysis, and execution.
Key Components of Structural Engineering
Within the domain of structural engineering, several key components define the activities and responsibilities of structural engineers.
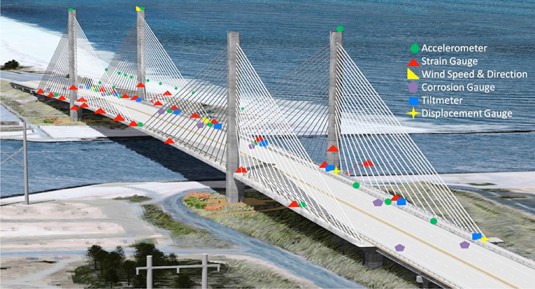
Structural Health Monitoring (SHM)
Structural health monitoring involves the continuous assessment of structural integrity and performance to detect potential defects, deterioration, or damage. Through the use of sensors, data acquisition systems, and advanced analytical techniques, engineers can monitor the condition of structures in real time and implement timely maintenance interventions to ensure their long-term viability.
Structural Safety
Ensuring the safety of structures and their occupants is paramount in structural engineering. Engineers assess structural safety by considering factors such as load-bearing capacity, stability, and resilience to various hazards, including seismic events, wind loads, and environmental factors. By adhering to rigorous safety standards and codes, engineers mitigate risks and enhance the overall safety of infrastructure projects.
Structural Analysis
Structural analysis forms the backbone of structural engineering. It enables engineers to predict and evaluate the behavior of structures under different loading conditions. Through mathematical modeling, computational simulations, and finite element analysis (FEA), engineers assess structural responses, including stresses, deflections, and deformations, to optimize designs, ensure structural integrity and comply with safety standards.
Structural Design
The design phase of structural engineering involves translating conceptual ideas into tangible structures that meet functional, aesthetic, and performance requirements. Engineers in structural design develop detailed designs, specifying dimensions, materials, and construction methods while considering factors such as sustainability, cost-effectiveness, and environmental impact, for different structural configurations.
Advanced structural design tools such as computer-aided design (CAD) software facilitate the creation of technical drawings and models, streamlining the design process and enhancing collaboration among project stakeholders.
The usage of software hugely enhances with opportunity to store, retrieve, and reuse structural configurations in digital format in what is now fashionable to call "digital thread".
Structural Engineering of Building Structures: A Short Review
The art and science of structural engineering have played a pivotal role in shaping the built environment throughout human history.
Building structures' design and construction processes have evolved significantly during the last millennia, driven by advancements in materials, technology, and engineering principles.
This article continues with a very short historical review, exploring the fundamental principles of why buildings stand up and why they fall, with strategies employed in structural design.
Understanding Structural Stability
The stability of a building structure is determined by its ability to withstand various forces and loads without collapsing. Structural engineers employ mechanics, physics, and materials science principles to analyze and design structures that balance these forces, ensuring stability, safety, and longevity.
Key factors influencing structural stability include the distribution of loads, the selection of appropriate materials, and the structural configuration.
Historical Overview
Throughout history, civilizations have demonstrated ingenuity in designing and constructing towering structures adapted to their environments and cultural contexts. Ancient civilizations such as Egyptians or Romans utilized stone, brick, and mortar to construct enduring structures such as pyramids, temples, and aqueducts. Those structures showed advanced engineering techniques and craftsmanship at an early stage.
During the Middle Ages, the development of Gothic architecture in Europe saw the emergence of towering cathedrals and churches characterized by soaring vaulted ceilings, flying buttresses, and intricate stone tracery. These structures exemplified the innovative use of load-bearing systems and pointed arches to achieve unprecedented heights and stability.
Types of Structures and Strategies in Civil and Environmental Engineering
From traditional vernacular architecture to modern marvels of engineering, building structures encompass a diverse range of typologies, each with its unique challenges and design strategies:
Low-Rise Residential Structures: Traditional dwellings such as cottages, cabins, and adobe houses employ simple structural systems based on load-bearing walls, timber framing, or earth construction techniques. These structures prioritize durability, thermal comfort, and cultural significance, often reflecting the local climate and available materials.
High-Rise Skyscrapers: Modern skyscrapers represent the pinnacle of structural engineering, utilizing advanced materials such as steel, reinforced concrete, and glass to achieve new heights and aesthetical expression. High-rise buildings employ innovative structural systems such as moment-resisting frames, shear walls, and diagrid structures to resist lateral loads and optimize vertical space utilization.
Bridges and Infrastructure: Structures extend beyond vertical constructions to include bridges, tunnels, and other infrastructure projects. Each type of structure presents unique challenges in terms of span, load distribution, and environmental conditions. Strategies for bridge design range from simple beam bridges and arch bridges to complex cable-stayed and suspension bridges, each optimized for its specific span and site conditions.

Case Studies - From the Igloo to the Skyscraper
The Igloo: The traditional Inuit igloo exemplifies ingenious structural engineering, utilizing compacted snow blocks to form a dome-shaped shelter that provides insulation, stability, and protection from harsh Arctic conditions. The circular form of the igloo distributes loads evenly, while the tapered design minimizes heat loss and enhances structural strength.
The Skyscraper: Iconic skyscrapers such as the Empire State Building or the Burj Khalifa showcase the evolution of structural engineering in the vertical direction. These structures employ advanced materials and construction techniques, including steel framing, reinforced concrete cores, and tuned mass dampers, to resist gravity, wind, and seismic forces while accommodating complex architectural programs.
Unveiling the Synergy: Structural Engineering, FEA, and Optimization
In civil engineering, structural engineering ensures infrastructures' stability, safety, and functionality. Finite Element Analysis (FEA) is a core tool: it is a powerful computational method that enables engineers to simulate and analyze complex structures under various loading conditions. In tandem with optimization techniques, FEA revolutionizes the design process, driving efficiency, innovation, and sustainability in structural engineering projects.
Understanding Structural Engineering
Structural engineering encompasses the science and art of designing and constructing buildings, bridges, and other infrastructure to withstand loads and environmental factors. Structural engineers employ principles of mechanics, materials science, and mathematics, balancing three factors: safety, functionality, and aesthetics.
Finite Element Analysis (FEA)
FEA is a numerical method used to solve complex engineering problems by dividing a structure into smaller, more manageable elements. These elements are interconnected at nodes, allowing engineers to simulate structural behavior and predict responses to applied loads.
By discretizing the structure and solving for displacements, stresses, and strains, FEA software provides insights and quantitative answers on the performance and reliability of designs.
The basic equation governing the behavior of a finite element in FEA software is:
[K] * u = f
where [K] is the stiffness matrix, u is the displacement vector, and f is the force vector.
Leveraging AI Technologies
The above [K] * u = f equation, is already an approximation within linear elasticity, where the material behavior is assumed to follow Hooke's law, and deformations are assumed to be small enough to maintain a linear relationship between stress and strain (compared to more complex formulations that consider nonlinear material behavior, geometric nonlinearity, or other effects like viscoelasticity or plasticity).
Already this equation could give rise to some questions.
It takes a fraction of a second to read it, but how to solve it?
The solution in terms of displacements u given a force and a stiffness matrix [K] is far from trivial, for a large number of matrix elements. This complexity arises due to the need to solve a system of linear equations, which can become computationally intensive as the size of the stiffness matrix increases. Additionally, factors such as the numerical methods used for solving the equations and the accuracy requirements of the solution can further contribute to the non-trivial nature of the problem.
One of the main elements to take into consideration is the shape of the object to be simulated with FEA since geometry influences the discretization, connectivity, integration, and boundary conditions of the finite elements to solve numerically. It could require from minutes to hours.
So, would it be possible to have a solution, with a fair degree of accuracy, in a fraction of a second, given the shape of the object described by a 3D CAD? Can this be assimilated into the problem of image recognition for a 3D object? Roughly speaking, a numerical, physics-driven topic based on the knowledge of physical laws AND object geometry is reduced to a numerical, data-driven topic based on the knowledge of data AND object geometry. More to come in later sections! Meanwhile, please keep in mind the relationship between AI and FEA is asymmetrical. FEA provides data to AI. AI in structural engineering provides a very efficient way to discriminate between different design solutions via a human engineer and design iterations, or with an optimization algorithm. The best final candidates for structural performance generated with AI algorithms are checked with high-fidelity solutions such as FEA and/or laboratory testing to validate the quantitative prediction of the best candidates for structural performance. Human intelligence is, therefore, always at work!
Design Process and Design Optimization
Optimization techniques play a crucial role in structural engineering, enabling engineers to refine designs, improve performance, and minimize material usage while meeting design criteria and constraints. From minimizing weight and cost to maximizing structural strength and energy efficiency, optimization algorithms offer powerful tools for achieving optimal design solutions tailored to the specific needs of each project.
Optimization seeks to find the best solution from a set of feasible alternatives, considering various objectives, constraints, and design parameters.
In structural engineering, optimization often involves maximizing structural performance, minimizing material usage, and optimizing cost-effectiveness while ensuring safety and regulatory standards compliance.
Generic Formulation of an Optimization Problem in Structural Engineering
Optimization problems in structural engineering can be formulated mathematically using objective functions, design variables, and constraints.
A general form of an optimization problem is:
minimize (or maximize) f(x) subject to: g(x) <= 0 h(x) = 0 lb <= x <= ub
Where:
- f(x) is the objective function representing the quantity to be optimized (e.g., structural weight, cost).
- g(x) and h(x) are inequality and equality constraints, respectively, representing design requirements and limitations.
- x is the vector of design variables representing parameters such as dimensions, material properties, and loads.
- lb and ub are lower and upper bounds on the design variables.
Example: Minimizing Material Usage in Truss Design
Consider the design of a truss structure subjected to specified loads and constraints. The objective is to minimize the total material usage while ensuring structural stability and strength. This optimization problem can be formulated as follows:
minimize Σ(w_i A_i) subject to: Σ(F_i) = 0 Σ(F_i d_i) = 0 A_min <= A_i <= A_max
Where:
- w_i is the weight density of each truss member.
- A_i is the cross-sectional area of each truss member.
- F_i is the force acting on each truss member.
- d_i is the distance from the centroid to the point of application of force for each truss member.
- A_min and A_max are the minimum and maximum allowable cross-sectional areas, respectively.
AI - Short Introduction for Users in Structural Engineering
Before talking about structural engineering AI, we need to talk about Artificial Intelligence.
Artificial intelligence (AI) has the potential to help traditional practices with its machine learning (ML) and deep learning (DL) declinations. This section explores Machine Learning and Deep Learning and their implications for structural analysis, design optimization, and predictive maintenance in engineering applications.

Machine Learning
Machine learning is a subset of artificial intelligence that focuses on developing algorithms capable of learning from data and making predictions or decisions, without explicit programming.
Thus, ML can extract patterns and insights from large datasets, enabling computers to generalize and adapt to new information without human intervention.
In structural engineering, ML algorithms can help automate repetitive tasks too.
By training models on historical data, engineers can develop predictive models that forecast structural behavior under different loading conditions, identify potential failure modes, and optimize designs for maximum performance and efficiency.
Key techniques in machine learning include:
Supervised Learning: In supervised learning, algorithms learn from labeled training data, where inputs are associated with corresponding outputs. This approach is commonly used for regression and classification tasks in structural engineering, such as predicting structural response or classifying structural elements based on material properties. This approach is particularly suited for AI in structural engineering since the physics-driven solvers (FEA) are surrogated by predictive models that operate a regression.
Unsupervised Learning: Unsupervised learning involves training models on unlabeled data to identify patterns or clusters within the data. In structural engineering, unsupervised learning techniques such as clustering and dimensionality reduction can be used to explore complex datasets, detect patterns, identify structural anomalies, and segment structural components based on similarities.
Reinforcement Learning: Reinforcement learning entails training agents to interact with an environment and learn optimal strategies through trial and error. While less common in structural engineering applications, reinforcement learning could be applied to tasks such as structural optimization and control, where agents learn to make informed decisions to maximize long-term performance objectives.
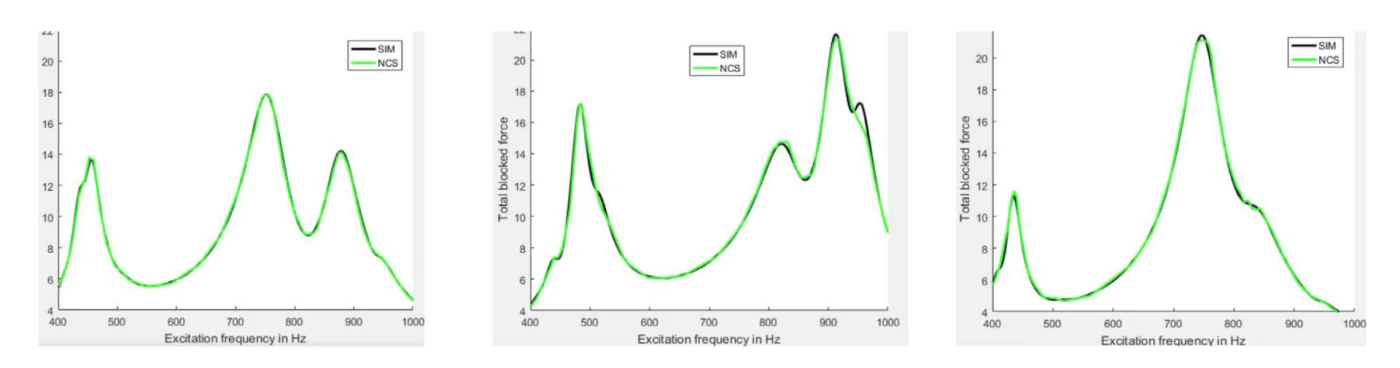
Deep Learning
Deep learning represents a most promising subset of machine learning that focuses on training artificial neural networks with multiple layers of interconnected nodes, known as neurons. Deep learning architectures, such as convolutional neural networks (CNNs) and recurrent NNs (RNNs), excel at capturing complex patterns and relationships in high-dimensional data, making them well-suited for tasks such as image recognition, natural language processing, and time-series analysis.
In structural engineering, deep learning offers opportunities for enhancing predictive modeling, anomaly detection, and decision support systems. By leveraging deep neural networks, engineers can analyze vast amounts of structural data, including sensor measurements, imagery, and simulation outputs, to gain deeper insights into structural behavior, detect potential defects or damage, and optimize maintenance strategies for infrastructure assets.
Key aspects of deep learning include:
Recurrent Neural Networks (RNNs): RNNs are designed to model sequential data with temporal dependencies. This makes them suitable for time-series analysis and sequence prediction tasks. In structural engineering, RNNs can be applied to tasks such as predicting structural response under dynamic loading conditions or forecasting maintenance requirements based on historical data.
Convolutional Neural Networks (CNNs): CNNs are particularly well-suited for analyzing spatial data such as images or sensor arrays. In structural engineering, CNNs can be used for tasks such as image-based defect detection, structural health monitoring, and automated feature extraction from sensor data.
Critique of AI in Structural Engineering
While artificial intelligence (AI) holds immense promise for revolutionizing structural engineering practices, it is essential to critically examine its limitations and challenges, particularly in terms of creativity, engineering expertise, and integration of AI in structural engineering.
One of the primary critiques of the AI approach in structural engineering is its potential to overshadow the role of human engineers and creativity in the design process. While AI algorithms excel at processing vast amounts of data and optimizing designs based on predefined objectives, they lack the contextual understanding of human engineers. The creative aspect of engineering design, based on experience, intuition, and domain expertise, cannot be fully replicated by AI systems alone.
FE Analysis and Analysis Process - AI in Structural Engineering.
Finite element (FE) analysis serves as a cornerstone in structural engineering, enabling engineers to simulate and analyze the behavior of complex structures under various loading conditions. While AI-driven approaches can enhance the efficiency and accuracy of FE analysis through automation and optimization, they must be carefully integrated into the analysis process to ensure the results' robustness, reliability, and interpretability. Engineers are in charge of evaluating the suitability of AI techniques for specific analysis tasks and validating their performance against established methodologies and standards.
Potential Risks and New Possibilities for Structural Engineers
The widespread adoption of AI in structural engineering brings both potential risks and new possibilities. On one hand, AI offers opportunities for innovation in design and efficiency in operations, enabling all engineers, even without a background in finite element analysis software, to tackle complex design challenges and enhance decision-making processes. However, the rapid advancements in AI technology also pose risks such as an overreliance on black-box models.
Precision and Accuracy Estimation of AI in Structural Engineering
The estimation of accuracy compared to traditional computational analysis methods such as CAE (Computer-Aided Engineering) remains a key passage in the implementation of deep learning surrogates for structural analysis. Metrics can provide insights into the performance of deep learning models as compared to CAE simulations. An example is the R-squared (R2) index, which measures the proportion of the variance in the output that is predictable from the input. Uncertainty quantification techniques can also be implemented.
Wrap-Up: Potential for AI Structural Engineering and Use Cases
We reviewed how to integrate Artificial Intelligence (AI) into structural engineering. All the phases of design, analysis, and optimization can be affected.
This integration should be approached critically eye to respect human expertise and creativity. AI offers automation and efficiency for companies, but it should complement rather than replace the insights and intuition of experienced humans who are in charge of the reliability and interpretability of AI results.
Before being delivered as a push-button App to all engineers, the validation of AI in structural engineering (FEA surrogates) remains a must for experts preparing the apps. However, it is facilitated with quantitative indices available, such as R2 and advanced uncertainty quantification techniques.