Stress Concentration: Mitigating Risk Factors in Design Engineering
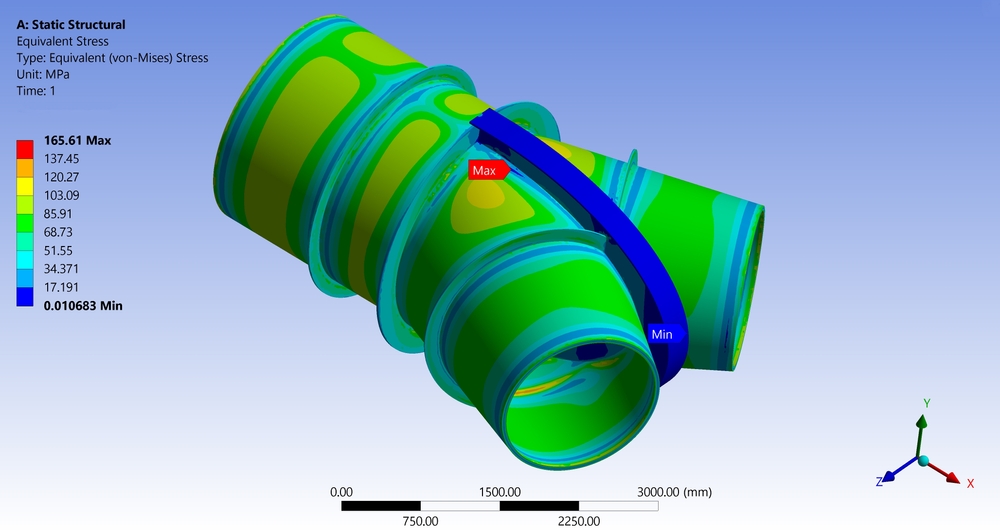
In this article, we will discuss how the study of stress concentration ensures the structural integrity and reliability of engineered components. We will also explore how simulation techniques ranging from FEA to deep learning applications can be implemented to assist iterative engineering design.
Stress concentration refers to localized areas within a material with higher stress levels than the average. Now, you may wonder why those concentrated stress areas occur. It can be due to geometric features, material defects, or the application of external forces (=loads). Ignoring stress concentration in the design process can have far-reaching and dramatic consequences, impacting the safety and performance of engineering systems and also the lives of vehicle passengers or civil structure inhabitants.
One of the key parameters used to quantify stress concentration is the stress concentration factor (K), which represents the ratio of the maximum stress at the point of interest to the nominal or applied stress. A stress concentration factor indicates a greater likelihood of failure in the vicinity of the stress concentration.
The stress concentration factor is dependent on the geometry of the part. Components with more significant discontinuities will have higher stress concentration factors. Thus, it is important to have tools estimating stress concentration factors via simulation or deep learning engineering prediction fed with stress concentration data.
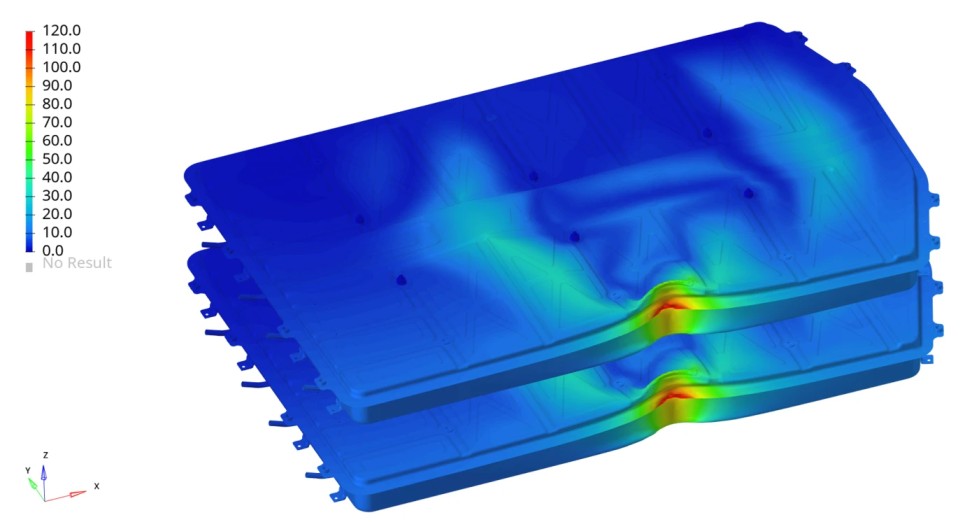
Neglecting stress concentration in design can lead to accidental damage and catastrophic failures. In mechanical components subjected to cyclic loading, such as turbine blades or automotive engine components, stress concentrations can accelerate fatigue crack initiation and propagation. Over time, stress concentration leads to unexpected component failures, posing serious safety risks and resulting in costly downtime or even accidents.
Furthermore, in structures subjected to dynamic loads, such as bridges or aircraft wings, stress concentrations can amplify the effects of vibrations and dynamic forces, leading to premature fatigue failure or structural instability. Failure to account for stress concentration effects in such applications can compromise the structural integrity of the entire system, endangering lives and causing substantial economic losses.
Industry Examples
In the aerospace industry, weight reduction is critical for fuel efficiency and performance. Designers often employ lightweight materials and complex geometries to achieve their goals. However, these design choices can introduce stress concentration sites in abrupt changes in geometry such as corners. Not addressing these stress concentrations can result in catastrophic failures during flight.
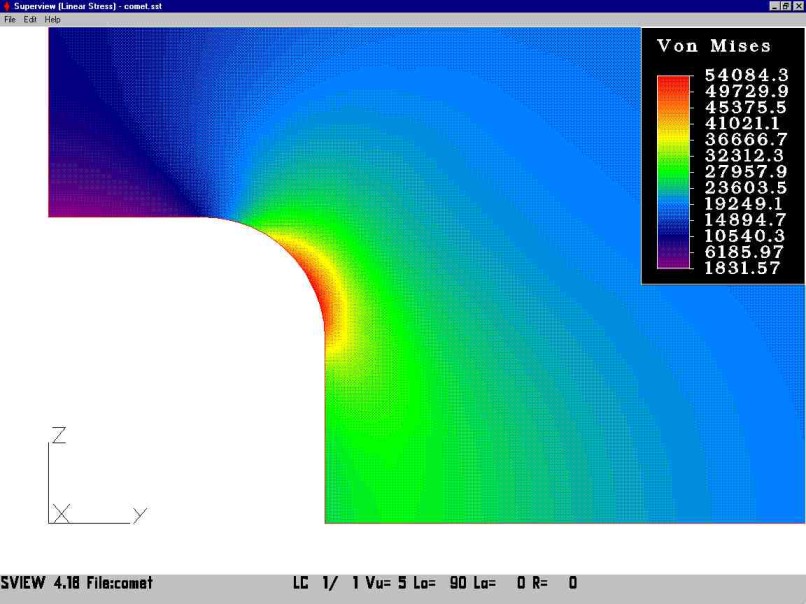
This highlights the potential beneficial impact of preemptive stress analysis (via FEA simulation, see below for more detail) to support mitigation strategies in aerospace design.
In the automotive industry, components such as suspension parts, chassis components, and drivetrain elements are subjected to varying loads and environmental conditions. Stress concentrations arising from manufacturing imperfections or design flaws can significantly reduce the fatigue life of these components, leading to unexpected failures on the road. Automotive manufacturers invest substantial resources in finite element analysis to identify and mitigate stress concentration effects during the design phase.
Finally, stress concentrations can also occur in electronic devices and microelectromechanical systems (MEMS), where miniaturization and high operating speeds are common requirements. In these applications, stress concentrations at interfaces or microstructural defects can lead to premature failure of electronic components, compromising the reliability and functionality of electronic devices due to accidental damage.
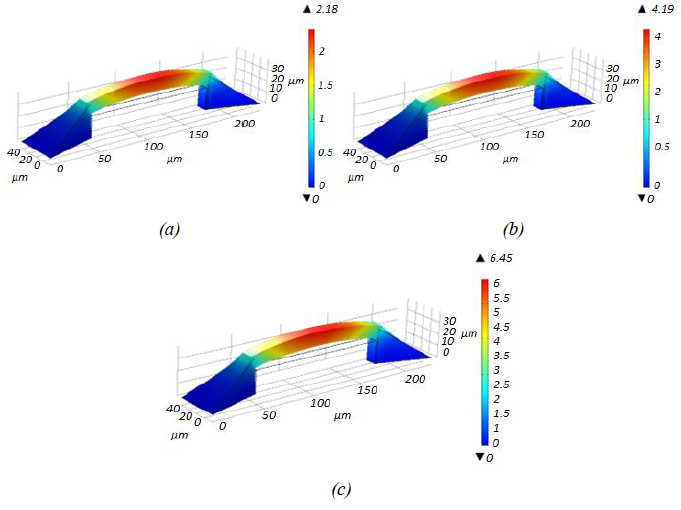
What Is Stress?
Stress is a fundamental concept in engineering mechanics, describing the internal forces experienced by a material under external loads. Stress concentration, on the other hand, refers to the localized increase in stress levels at specific points within a material due to geometric irregularities.
Mathematically, since it refers to the internal force per unit area experienced by a material when subjected to external loads or deformation, stress (σ) is defined as the force (F) applied to a material divided by the cross-sectional area (A) over which the force (F) is applied:
σ = F / A

Stress Forms
Stress can manifest in different forms depending on the type of load and the deformation experienced by the material.
The three primary types of stress are tensile, compressive, and shear stress:
- Tensile Stress - It occurs when a material is pulled or stretched along its length. Tensile stress is expressed as a positive value.
- Compressive Stress - Compressive stress occurs when a material is subjected to forces that compress or shorten it along its length. Compressive stress is characterized by a decrease in length and is typically expressed as a negative value.
- Shear Stress - Shear stress arises when forces are applied parallel to the material surface, causing one layer to slide relative to another layer. Shear stress is characterized by deformation or distortion of the material along the plane of applied force.
Knowing the distribution and magnitude of stress within a material is essential for ensuring the structural integrity and safety of engineering components. However, stress is not uniformly distributed across a material, especially with geometric irregularities or discontinuities. This non-uniform distribution of stress can lead to localized areas of significantly higher stress levels known as stress concentrations.
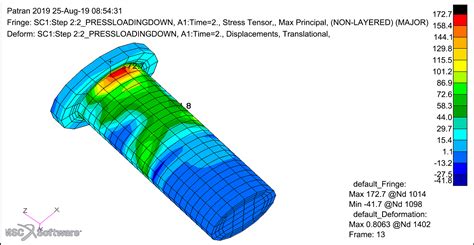
Stress Concentration
Stress concentration occurs when there's a localized increase in stress within a material due to the presence of geometric features, such as sharp corners, holes, or changes in cross-sectional area. These geometric irregularities disrupt the smooth flow of stress through the material, causing it to concentrate at specific points.
Stress concentrations significantly influence the overall mechanical behavior of a structure or component. The stress concentration factor K says how much stress is amplified at the point of interest compared to the applied or nominal stress. A higher stress concentration factor indicates a greater risk of failure at the highest stress point.
With the relationship between stress and stress concentration, you can identify critical areas prone to failure and implement appropriate design modifications or mitigation strategies to enhance the reliability and performance of engineered systems.
Incorporating stress concentration factor analysis into the design process enables engineers to optimize component geometry, select appropriate materials, and minimize the risk of unexpected failures.
Importance of the Stress Concentration Factor in Design Engineering
We will discuss the broader implications of neglecting stress concentration in design and explain how it can impact overall system performance.
Stress Concentration Effect
In this section, we will explore the stress concentration effects on materials and structures. We will focus on specific aspects such as high-stress concentration, fatigue strength, and fatigue crack. By examining these factors in detail, we can gain insights into the challenges posed by stress concentration and the strategies to mitigate its adverse effects.
Understanding the effects of stress concentration is paramount for engineers and designers as it directly impacts the safety, reliability, and performance of engineered systems. By identifying stress concentration, engineers can develop better designs that withstand the challenges of real-world applications. Let us now explore the stress concentration and its implications for materials and structures.
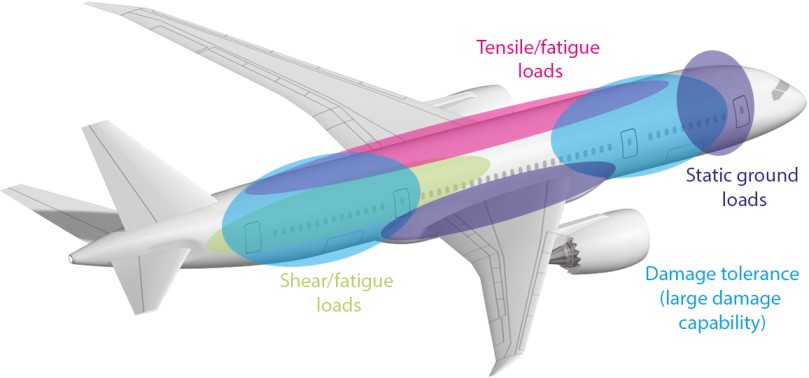
Stress Concentration Factors
Stress concentration factors (SCFs) are numerical values that quantify the extent to which stress is amplified at a specific point due to geometric irregularities or loading conditions.
Mathematically, the stress concentration factor can be expressed as:
K = σ_max / σ_nominal
Here, K denotes our stress concentration factor while σ_max represents the maximum stress and σ_nominal denotes the applied or nominal stress in the gross cross-section.
Stress concentration factors play a crucial role in design engineering by providing insights into areas of potential weakness in a structure. SCFs can be categorized based on the types of geometric features or loading conditions that contribute to estimating stress concentration factors, including:
Geometric Features: The stress concentration factor is associated with geometric features such as sharp corners, holes, fillets, notches, and changes in cross-sectional area.
Loading Conditions: The stress concentration factor is related to different loading conditions, such as tension, compression, bending, torsion, and combined loading.
Understanding these types of stress concentration factors allows engineers to assess the potential risks associated with specific design features or loading scenarios and implement appropriate mitigation strategies.
High Values of Stress Concentration Factor
High-stress concentration occurs when the stress levels at a localized point within a material significantly exceed the average stress. This is particularly significant in design engineering because it can lead to premature failure of components or structures. Recognizing and addressing a high-stress concentration factor is essential for designing robust and reliable structures that can withstand the expected operating conditions without experiencing premature failure.
Aspects contributing to a high-stress concentration factor include geometric discontinuities such as sharp corners, notches, holes, and sudden changes in geometry that can be created when stress concentrations occur at concentration points.
Material defects are also important. They include inclusions, voids, and other material imperfections. Extreme or fluctuating applied loads can further exacerbate the stress concentration factor, especially in dynamic applications.
Fatigue Strength
Fatigue strength refers to the ability of a material to resist cyclic loads and withstand repeated stress cycles without failure. Understanding fatigue strength is crucial because stress concentrations can accelerate the initiation and propagation of cracks. Factors affecting fatigue strength include:
- Stress Concentration Effects: High-stress concentrations can lead to localized plastic deformation, crack initiation, and eventual fracture under cyclic loads.
- Material Properties: The fatigue strength of a material depends on its microstructure, grain size, heat treatment, and other factors.
- Load Conditions: The magnitude, frequency, and duration of loading influence the fatigue behavior of materials.
Fatigue Cracks
Fatigue cracks are cracks that initiate and propagate in a material subjected to cyclic loading, leading to eventual failure. Stress concentrations may play a critical role in forming and propogating cracks by locally increasing the stress levels above the material's endurance limit. Once initiated, cracks can propagate rapidly, compromising the integrity of the structure. Factors influencing fatigue crack propagation include:
- Stress Intensity: The magnitude of stress concentration determines the severity of stress intensity at the crack tip, influencing crack growth rates.
- Material Properties: The material resistance to fatigue crack propagation depends on its fracture toughness, fatigue crack growth rate, and other properties.
- Environmental Factors: Factors such as temperature, humidity, and corrosive environments can accelerate fatigue crack propagation.
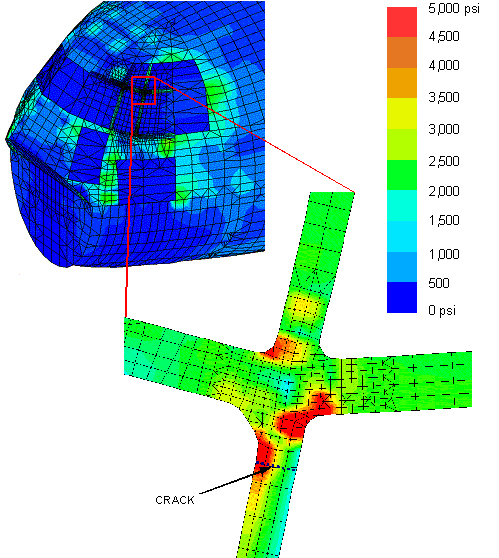
Stress Intensity Factors
The stress intensity factor is the magnitude of the stress singularity at the tip of a mathematically sharp crack in a linear elastic material. Each mode of fracture has an associated stress intensity factor. The illustrated "Mode-I", "Mode-II", and "Mode-III" stress intensity factors are defined for the opening, sliding, and tearing modes that are the main modes of fracture propagation.
How to Reduce Stress Concentrations
Let's explore methods and strategies for mitigating risk factors associated with stress concentration in design engineering, aimed at reducing stress concentrations and improving structural integrity.
Working on shape change, materials, and load conditions, and with advanced analysis techniques such as CAE and AI, engineers can mitigate the adverse effects of stress concentration and ensure the long-term performance of engineered systems. Geometric (shape) optimization involves refining the geometry of components to alleviate stress concentration at critical points, such as abrupt corners, notches, or holes. By smoothing shape transitions and eliminating abrupt changes, we can achieve a more uniform stress distribution, thus reducing the likelihood of failure.
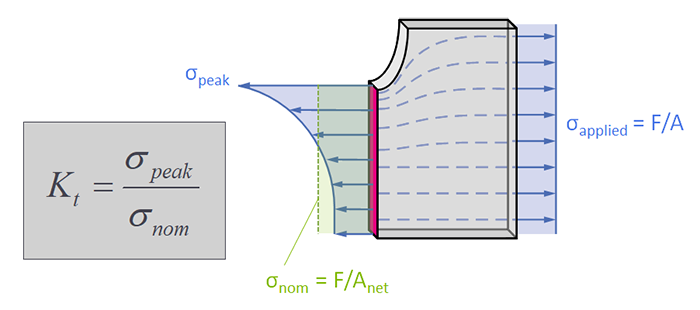
Another crucial aspect is material selection, where engineers choose materials with appropriate mechanical properties and resistance to stress concentration effects. By selecting materials with high fracture toughness and fatigue resistance, engineers can mitigate the impact of stress concentration on structural performance.
Load redistribution is another effective strategy. By redistributing applied loads or introducing load-relieving features, engineers can achieve more balanced stress distributions and reduce the risk of localized failure.
Structural redesign may also be necessary to eliminate or mitigate stress concentration features. This approach involves revisiting the design of components or structures to incorporate smoother transitions, fillets, or other stress-relieving features.
Finite Element Analysis (FEA) plays a crucial role in stress analysis by providing engineers with detailed insights into stress distribution and reducing stress concentration. Through advanced numerical simulations, engineers can accurately predict stress levels and identify areas prone to stress concentration, enabling informed design decisions. Furthermore, fatigue analysis is essential for assessing the mechanical life of components subjected to cyclical loading. By evaluating fatigue strength and identifying potential fatigue failure modes associated with stress concentration, engineers can design components with sufficient durability to withstand real-world operating conditions.
Check our blog for examples of deep learning applications.
Advanced Techniques in Design Engineering: FEA and AI
FEA works by discretizing a complex structure into smaller, more manageable elements, allowing engineers to approximate its behavior through numerical simulation. It's a physics-informed computer approach based on the formulation of governing equations that describe the behavior of each element and their interactions within the structure. FEA relies on the principle of equilibrium, where the sum of internal forces within each element equals the ratio of the highest external loads applied to the structure. This principle is expressed through equilibrium equations, typically represented as:
∑F_internal = ∑F_external
where: ∑F_internal = sum of internal forces within each element and ∑F_external = the sum of external loads applied to the structure.
By solving these equations iteratively across all elements, FEA vizualizes in 3D its mechanical response, including stress distribution, deformation, and displacement.
Can deep learning assist engineers? By using previous FEA analyses and associated CAD geometries, it can provide an intuitively deployable solution available to all engineers and not only FEA specialists interested in mitigating risk factors in design.
FEA and Stress Concentration Factors
Stress concentration is readily identified through FEA or Deep Learning surrogates. By analyzing stress distribution across the structure, engineers can pinpoint areas where stress concentrations occur more than the stress intensity factor of the surrounding material. As we have seen, these localized regions of high stress often coincide with geometric irregularities where stress tends to accumulate.
FEA allows engineers to quantify stress concentration through the stress concentration factor (K), which represents the ratio of maximum stress at a specific point to the applied or nominal reference stress amount. By calculating the stress concentration factor with FEA, engineers can assess the severity of stress concentration and prioritize design modifications with strategies such as geometric optimization, filleting, and material selection.
As a next step in the future with AI, thanks to deep learning deployment, any engineer can be informed by surrogates of FEA results to minimize the stress concentration factor and enhance structural integrity.
Reference Stress Book for Designers:
Walter D. Pilkey, Deborah F. Pilkey, Zhuming Bi: "Peterson's Stress Concentration Factors", DOI:10.1002/9780470211106